Atmosphere emission sources includes oxygen molecules,
carbon dioxide, water vapor, droplets of water,
dust aerosols and
sulfuric acid aerosols (polar stratospheric cloud droplets). At
frequencies where the MTP operates, and at the high altitudes where the
MTP is usually flown, the only emission source to consider is oxygen
molecules. Flight at lower altitudes, where clouds are present, would
have to include liquid water droplets.
Oxygen
The MTP operates at frequencies where oxygen molecules interact with
microwaves. Oxygen molecules rotate with energies that differ by
amounts that correspond to the energy of microwave photons in the
frequency range 48 to 71 GHz (and at 119 GHz). At sea level the
typical oxygen molecule is distorted so
much from recent collisions that a molecule's change in energy as it
switches from one rotational state to another can differ from the low
pressure energy difference, and this causes a "pressure broadening" of
the resonance frequency. As a result of pressure broadening the oxygen
molecule absorption spectrum has a structure that variers significantly
with altitude, as
the following graph
illustrates.
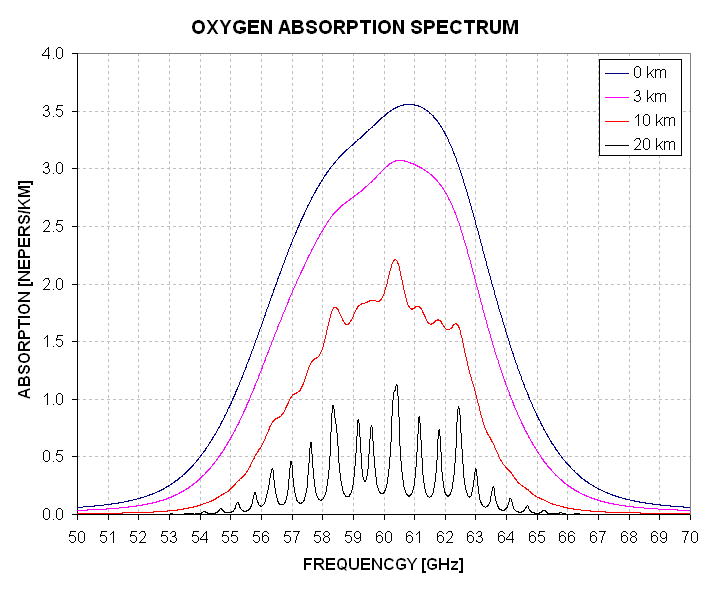
Figure 5.1 Oxygen molecule absorption spectrum for 4 altitudes
(using US Standard Atmosphere temperatures and pressures).
Absorption coefficient, Kv, can be given in units of Nepers per
kilometer [Neper/km]. To convert to [db/km] multiply the [Neper/km]
value by
4.343. In a medium where Kv = 1 [Neper/km] a photon will have a 1/e =
0.37 probability of being transmitted 1 km (i.e., without being
absorbed). It will also have a 1 - 1/e = 0.73 probability of being
absorbed. Every
medium that absorbs photons also emits them, and oxygen molecules are
the main source of this thermal radiation at frequencies near 60 GHz.
Carbon Dioxide
Carbon dioxide molecules don't interact with photons having frequencies
below 115 GHz, so they can be ignored for the MTP.
Water Vapor
Water vapor molecules have a resonate absorption features at 22.2 GHz
and 183 GHz, as well as a poorly understood non-resonant component at
microwave frequencies. Water vapor has a distribution versus altitude
characterized by a much smaller scale height than dry air. If relative
humidity were constant with altitude then water vapor would have a
scale height of ~2 km instead of the dry atmosphere's 8 km, and in fact
this 2 km scale height for water vapor is indeed found to hold quite
often. Because most water vapor molecules are found near the
surface their presence at typical MTP altitudes can be ignored. For
those few occasions that an MTP is operated within the planetary
boundary layer it is still acceptable to ignore water vapor absorption
since at these low altitudes the level of oxygen molecule absorption is
still much higher. Nevertheless, here is the water vapor absorption
spectrum near
sea level.
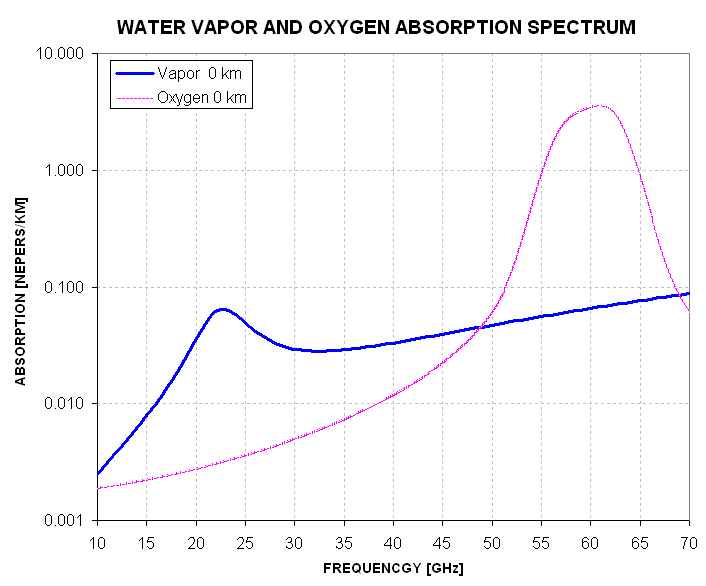
Figure 5.2 Water vapor absorption for typical sea level
conditions in relation to oxygen absorption. An MTP operates at
frequencies in the 55 to 60 GHz region.
Considering that the MTP operates at frequencies near the peak for
oxygen absorption (55 to 60 GHz) when an MTP flies at low altitudes the
additional absorption of the atmosphere due to water vapor is small in
relation to that of oxygen molecules, being approximately 50 times
smaller. At normal flight altitudes the water vapor contribution is
even smaller due to the difference in scale heights for water vapor and
oxygen.
The semi-empirical equation for water vapor absorption is given by
Waters (1976):
Kv [Neper/km] = V * W * F2 * T
-3/2 * { (7.18 * 10 5 e-644/T ) / (T
* X) + 2.77 * 10 -3 }
where W [GHz] = 2.46 * (P / 1013) * (
300 / T ) 0.626 * ( 1 + 0.018 * ( V * T ) / P )
and X [GHz2]
= ( 494.4019 - F 2 ) + ( 2 * F * W )
V
[gm / m3] = water vapor density
P [mb] = air pressure
T [K] = air temperature
F [GHz] = frequency
Notice that there's a "resonance" feature at SQRT(494.4019) = 22.235
[GHz]. The "line width parameter" W accomodates the fact that
high pressure broadens the resonant absorption feature, which causes
absorption at line center to decrease while farther than ~1.63 GHz from
line center absorption increases. At MTP frequencies (53 to 60 GHz)
colder means more absorptive, and lower pressure means lower
absorption. However, changes in altitude correspond to water vapor
mixing ratio changes that are more important than pressure or
temperature changes, as the following graph shows.
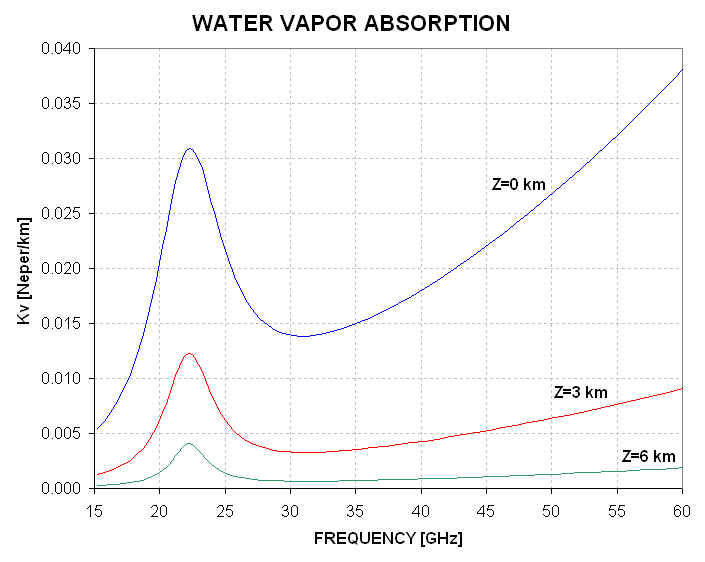
Figure 5.3 Water vapor absorption for
typical conditions at 3 altitudes (1000, 700 and 300 mb).
Since water vapor mixing ratio (and density) decreases with a scale
height of about 2 km small increases in altitude correspond to large
decreases in water vapor absorption. At tropospheric altitudes (10 to
16 km) water vapor absorption can be ignored.
Cloud Liquid Water Droplets
Most cloud liquid water drops are small compared to microwave
wavelengths. Only large rain drops are comparable in
circumference to wavelength, which means that cloud drops absorb in a
way that is not influenced by drop size. This is good, because it means
that clouds absorb in proportion to
their average volume liquid water content, expressed in units of grams
per cubic meter. The term "liquid water content" (LWC) is commonly
used. A typical cloud near the surface has LWC = 0.5 [g/m3]. Here's the absorption spectrum for such a cloud.
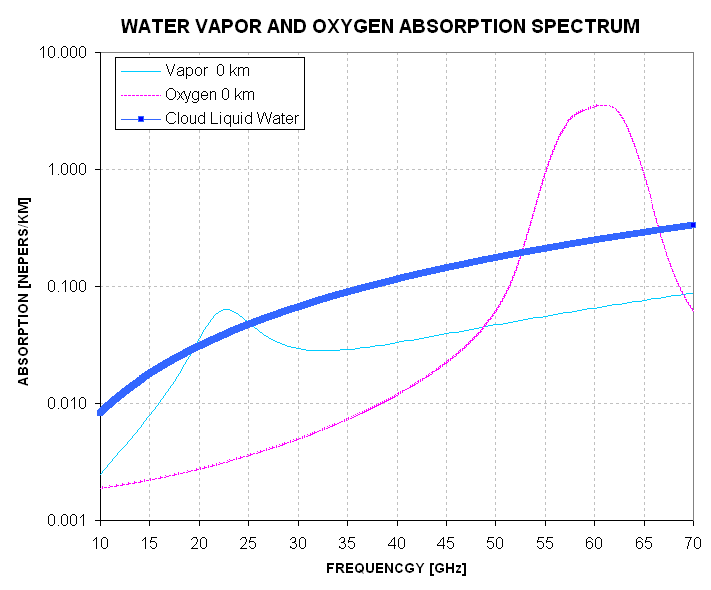
Figure 5.4 Liquid water (cloud)
absorption for typical sea level
conditions in relation to oxygen absorption. The cloud is
assumed to have LWC = 0.5 [g/m3] and a temperature of 288 K.
This graph shows that within a typical cloud layer (at low
altitude) the liquid water droplets absorb more MTP photons per unit
distance than water vapor, though less than oxygen. Keep in mind that
this compononet of absorption is confined to the cloud layer (which
might be 1 km) whereas the other two components extend over a much
larger altitude region. For an MTP flying in the boundary layer
absorption by oxygen will exceed that for any other component by 1 to 2
orders of magnitude.
Cumulus clouds have low LWC values, typically
0.1 [g/m3],
which produce an absorption spectrum 4 times lower than in the above
graph. Only stratus clouds have large LWC values, approaching 1.0 [g/m3].
Clouds that are colder than freezing are either a mixture of water and
ice (in the temperature region 0 C to -40 C) or pure ice crystals
(colder than -40 C). Pure ice crystals are nearly transparent at MTP
frequencies. A typical cirrus cloud consisting of only ice crystals
would have to be ~400 km thick to produce one Neper of absorption at 60
GHz. Therefore, cirrus clouds (most of which are pure ice crystals) can
be ignored by MTP users.
The equation for liquid water absorption is:
Kv [Np/km] = 0.075 * LWC
[g/m3] * (280 / T[K]) 6.87
* (F / 22.2 [GHz]) 1.9
where F is frequencey [GHz]. This equation shows that cold clouds are more absorptive than warm ones. For
every 10 K of additional coldness the liquid water absorption increases
~28%. Note the exponent for the frequency dependence, 1.9. This
exponent would be 2.0 if cloud droplets were all much smaller than
wavelength. Since some droplets are comparable to wavelength, the 1.9
exponent provides a better empirical fit to real-world clouds. Cloud
droplets are poor scatterers at MTP wavelengths (because of their small
size), and this component of "loss" can be ignored. Rain drops can scatter at the few
tens of percent of ozygen absorption at 60 GHz. Even for these
conditions liquid water absorption is more important than scattering.
Aerosols
Non-water aerosols consist of dust and smog (at low altitudes), which
are unimportant at MTP frequenceis. For example, a dust storm with an
optical scattering loss having an e-folding distance of 500 meters will
produce a only a 0.3 % loss per 500 meters at MTP frequencies (assuming
100 micron diameter aerosols). Oxygen absorption is 2 to 3 orders of
magnitude greater. Smog does not have absorption features at microwave
frequenceis (the important ones are at higher frequencies).
At high altitudes (in the lower stratospehre) the only aerosols that
can possibly affect MTP absorption are small droplets of sulfuric acid,
liquid water and nitric acid (polar stratospheric). The Type I PSC
aerosols consist of nitric acid tri-hydrate (NAT) particles ~1 or 2
microns in diameter. They are found where temperatures are colder than
195 K and where N2O mixing ratios are high (i.e., above the
tropopause). When temperatures drop below 190 K water vapor swells the
NAT aerosols, producing Type II PSCs. These aerosols have diamters of 5
to 20 microns (the larger ones have fall rates that "de-nitrify" the
layer with large Type II aerosols). I have calculated that Type I and
Type II PSC aerosols are not detectable through their absorption
effects at 60 GHz. Scattering by these aerosols is also too small for
detection. Volcanic clouds have two components, ash (dust) and sulfuric
acid, and typically they will be together as a droplet of sulfuric acid
with an ash grain inside. These aerosols produce negligible absorption
and scattering compared with that of oxygen molecules. Condensation
nuclei are all too small to affect microwaves.
This concludes a survey of potential atmospheric absorbers at MTP
frequencies. The "message" presented is a reassuring one, namely, only
oxygen molecular absorption needs to be considered for an MTP flying
above the planetary boundary layer. The next chapter makes this
assumption as we begin to explore ways to retrieve temperature profiles
from MTP observables.
Go to Chapter #6
(next chapter)
This is Chapter 5
Go to Chapter #4 (previous chapter)
Return to Introduction
____________________________________________________________________